Crater Lake Area Field Trip: The Geology of Crater Lake National Park
by Howell Williams
Cover
State of Oregon
LUNAR GEOLOGICAL FIELD
CONFERENCE GUIDE BOOK
PREPARED BY
- V. Peterson and E. A. Groh-Editors
- J. Newhouse-Cartographer
and the Editorial Staff of the
State of Oregon Department of Geology and Mineral Industries
STATE GOVERNING BOARD
Frank C. McColloch, Chairman . . . Portland
Harold Banta . . . . . . . . . . Baker
Fayette I. Bristol . . . . . . . Grants Pass
Hollis M. Dole
State Geologist
Conference Sponsored by
The University of Oregon Department of Geology and The New York Academy of Science
August 22 to 29, 1965 Bend, Oregon, U.S.A.
Second Edition
1966
SCHULTZ-WACK-WEIR, Inc.
Publishers
Portland, Oregon, U.S.A.
Crater Lake Area Field Trip
THE GEOLOGY OF CRATER LAKE NATIONAL PARK, OREGON*
With a Reconnaissance of the Cascade Range Southward to Mount Shasta
By Howel Williams **
Abstract
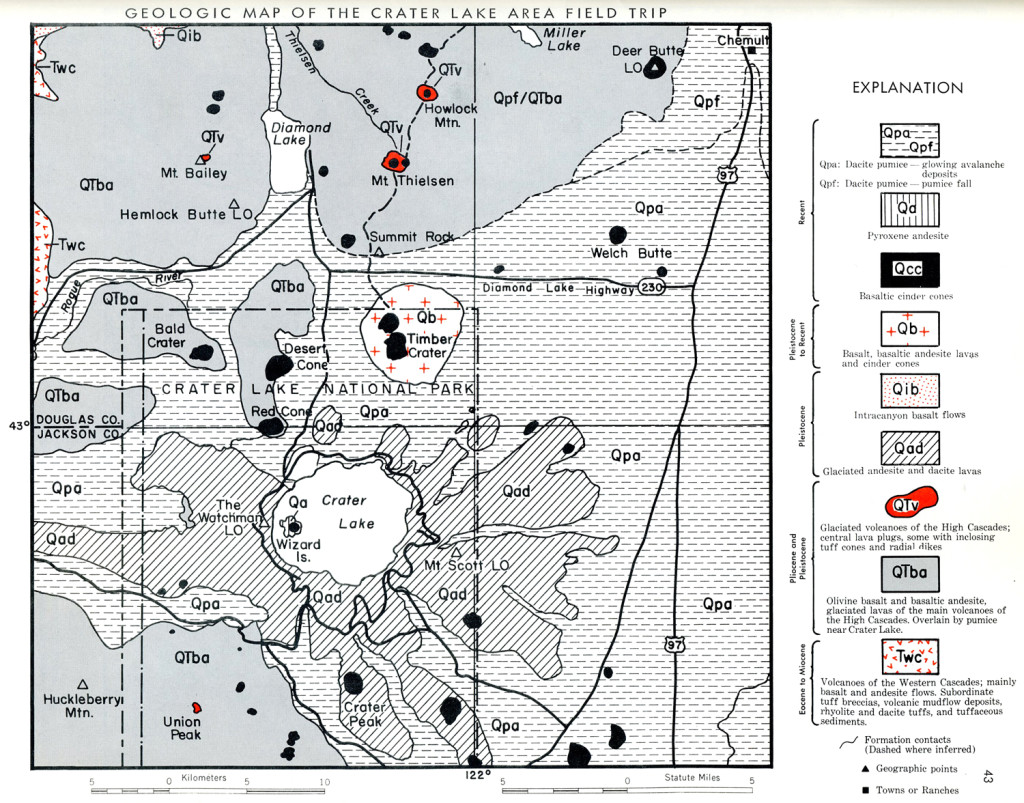
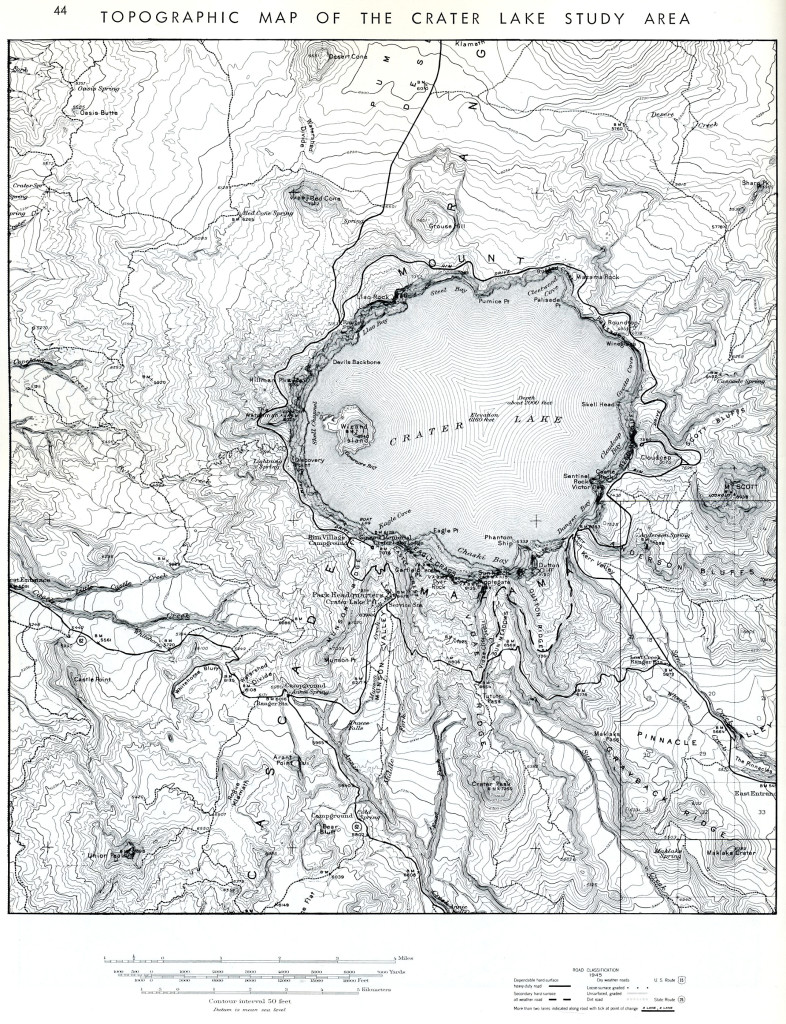
Accordingly, 11.75 cubic miles (49 km 3) of ejecta were laid down during these short-lived eruptions. In part, it was the rapid evacuation of this material that withdrew support from beneath the summit of the volcano and thus led to a profound engulfment. The collapse was probably as cataclysmic as that which produced the caldera of Krakatau in 1883.Some process other than the expulsion of magma from the feeding chamber must also have operated. Whereas 17 cubic miles (70 km3) of the volcano disappeared, at most 11.75 cubic miles (49 km3) of ejecta were laid down. Moreover, by far the bulk of these ejecta consists of vesicular glass. The equivalent volume of liquid magma was less than half as much. Accordingly, it must be concluded that during or immediately before the great eruptions, large volumes of magma were injected into fissures at depth. It was by a combination of deep-seated intrusion and explosive eruption that the magma chamber was drained to make room for the collapse of the peak of Mount Mazama. Doubtless the caldera floor also subsided by cooling and solidification of the magma left below.The collapse of Mount Mazama was eccentric with respect to the former summit, for this lay well to the south of what i s now the center of Crater Lake. This eccentric collapse was controlled by the preexisting semicircular line of weakness along which the Northern Arc of Vents was formed.The formation of Crater Lake took place approximately 5000 years ago. The discovery of artifacts beneath the pumice deposits shows that man already inhabited this part of Oregon and was a distant witness of the catastrophe.After a period of quiet of unknown duration, activity commenced anew. Close to the western edge of the caldera rose the cone of Wizard Island, the final act of which was to erupt a rugged sheet of blocky lava, perhaps no more than a thousand years ago. As intra-caldera eruptions went on, rain and snow formed a lake on the floor, and this continued to gain in volume until it reached a depth of almost 2000 feet (610m).
Note by the Editors
Since the time Williams published this work on the geology of Crater Lake National Park, radiocarbon dating of charcoal incorporated in the pumice flows revealed an age of about 6600 years for the eruption. This age has been further confirmed by radiocarbon dating of archeological sites at which Mazama ash was identified (Libby 1952; Rubin and Alexander 1960).
Recent studies on the distribution of Mazama ash show that it extends at least 600 miles (970 km) northerly from its source and more than 500 miles (800 km) to the east. This corresponds to a minimum area for the ash fall of about 350,000 square miles (900,000 kmZ) (Powers and Wilcox 1964; Fryxell 1965).
A detailed bathymetric survey of Crater Lake was made in 1959 by the U.S. Coast and Geodetic Survey. Byrne (1962) prepared a bathymetric chart from the survey data and described some of the underwater features. His chart and text are reprinted herein.
References
Benson, G. T., 1965, The age of Clear Lake, Oregon: Oregon Dept. Geology and Mineral Industries, The ORE BIN, v. 27, no. 2, p. 37-40.
Bullord, F. M., 1962, Volcanoes, in history, in theory, in eruption: Austin, Texas, Univ. of Texas Press.
Byrne, J. V., 1962, Bathymetry of Crater Lake, Oregon: The ORE BIN, v. 24, no. 10, p. 161-164.
Cotton, C. A., 1952, Volcanoes as landscape forms: New York, John Wiley &Sons, Inc., first ed. rev.
Fryxell, Roald, 1965, Mazama and Glacier Peak volcanic ash layers; relative ages: Science, v. 147, no. 3663, p. 1288-1 290.
Hampton, E. R., 1964, Geologic factors that control the occurrence and availability of ground water in the Fort Rock basin, Lake County, Oregon: U.S. Geol. Survey Prof. Paper 383-8.
Libby, W. F., 1952, Radiocarbon dating: Chicago, Ill., University of Chicago Press.
Nichols, R. L., 1938, Fissure eruptions near Bend, Oregon (abst.): Geol. Soc. America Bull., v. 49, no. 12, pt. 2, p. 1894.
_____, 1940, The flow mechanism of basalts, vol. 1: Cambridge, Moss., Harvard Univ. doctoral dissertation (unpub.).
_____, 1940, A lava-fan near Bend, Oregon (abst.): Am. Geophys. Union Trans. of 1941, p. 357-358.
_____, 1941, The velocity of the Big Obsidian Flow, Bend, Oregon (abst.): Am. Geophys. Union Trans. of 1941, p. 504-505.
_____, 1941, Tree rings in lava (abst.): Geol. Soc. America Bull., v. 52, no. 12, pt. 2, p. 1926.
Peterson. N. V.. and Groh. E. A,. 1961. Hole-in-the-Ground: The ORE BIN, v. 23, no. 10, P. 95-100.) 1963,. Recent volcanic landforms in central Oregon: The ORE BIN, v. 25, no. 3, p. 33-45.
_____, 1963, Maars of south-central Oregon: The ORE BIN, v. 25, no. 5, p.73-88.
_____, 1964, Diamond Craters, Oregon: The ORE BIN, v. 26, no. 2, p. 17-34.
_____, 1964, Crock-in-the-Ground, Lake County, Oregon: The ORE BIN, v. 26, no. 9, p. 158-166.
Powers, H. A., and Wilcox, R. E., 1964, Volcanic ash from Mt. Mazama (Crater Lake) and from Glacier Peak: Science, v. 144, no. 3624, p. 1334-1336.
Rittman, A., 1962, Volcanoes and their activity: New York, lnterscience Publishers, John Wiley & Sons, Inc.
Rubin, Meyer, and Alexander, Corrinne, 1960, U.S. Geol. Survey radiocarbon dates: Am. Jour. Sci. Radiocarbon Supplement, v. 2, p. 129-185.
Taylor, Edward M., 1965, Recent volcanism between Three Fingered Jack and North Sister, Oregon Cascade Range: The ORE BIN, v. 27, no. 7, p. 121-147.
Wentworth, C. K., and MacDonald, G. A., 1953, Structures and forms of basaltic rocks in Hawaii: U. S. Geol. Survey Bull. 994.
Williams, Howel, 1932, The history and character of volcanic domes: Univ. Calif., Pub., Dept. Geol. Sci. Bull., v. 21, p. 51-146., 1933, Mount Thielsen, a dissected Cascade volcano: Univ. Calif. Pub., Dept., Geol. Sci. Bull., v. 23, p. 195-214.
_____, 1935, Newberry volcano of central Oregon: Geol. Soc. America Bull., v. 46, p. 253-304.
_____, 1941, Calderas and their origin: Univ. Calif. Pub., Dept. Geol. Sci. bull., v. 25, p.239-346.
_____, 1942, The geology of Crater Lake National Park, Oregon: Carnegie Inst. Washington Pub.540.
_____, 1944, Volcanoes of the Three Sisters region: Univ. Calif. Pub., Dept. Geol. Sci. Bull., v. 27, p. 37-84.
_____, 1953, The ancient volcanoes of Oregon: Oregon State System of Higher Education, Condon Lectures, 2nd ed.
_____, 1957, Geologic mop of the Bend quad., Oregon, and a reconnaissance geol . mop of the central portion of the High Cascade Mts.: Oregon Dept. Geology and Mineral Industries.
_____, 1961, The floor of Crater Lake, Oregon: Am. Jour. Sci., v. 259, p. 81-83.